Quantum materials: Theoretical, experimental and computational physicists develop the technology of the future
Binghamton’s graduate program in physics is young but mighty, and home to transformative research
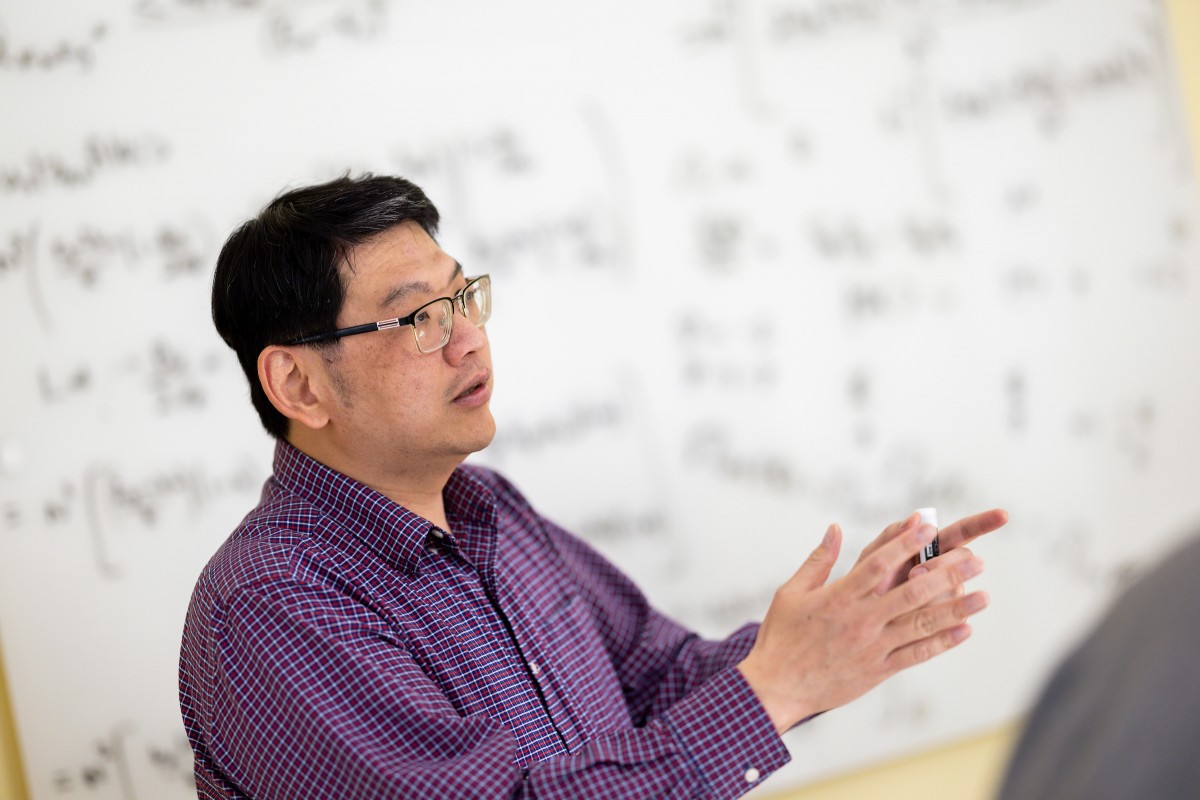
Look at a flat substance on the atomic scale, and you will find a strange new world that bears little resemblance to what you see with the naked eye.
“When you look at an atomic level, a material isn’t perfectly flat with perfect edges,” explained Binghamton University Associate Professor of Physics Pegor Aynajian. “It can be very rough, with atomic terraces that go in different directions, different edges, and defects.”
Aynajian works in a lab that is cleaner and colder than outer space, conditions that allow researchers to study exotic materials known as magnetic topological insulators at the atomic level. Known as quantum materials, they are at the frontier of condensed matter physics and may someday lead the way to technological innovations worthy of science fiction.
Topological materials and superconductors are among the research lines in Binghamton’s physics program, which encompasses theoretical, computational and experimental physics.
The initial graduate program ended in the 1980s and was relaunched in 2010, said Professor Alexey Kolmogorov, who joined the department in 2012. Since its revival, the program has become stronger every year and made U.S. News and World Reports’ best graduate programs rankings in 2023.
Faculty routinely receive research funding from the National Science Foundation, including Kolmogorov, whose work has been funded every year since 2014, and Aynajian, who received a $534,746 NSF grant in July. In the past 10 years, the physics department has secured three NSF CAREER awards and more than five NSF and Department of Energy grants, as well as a Department of Defense Multidisciplinary University Research Initiatives (MURI) grant.
Today, the program has around 45 graduate students and the department continues to hire new faculty members. Alumni have gone on to work at Google and Intel, in national labs and in academia. Physics undergraduates also participate in research and have even co-authored papers, which has led them to some of the best doctoral programs around the country. It’s an impressive record for a young program, faculty acknowledged.
Some Binghamton physicists work in high-tech labs, while others create computer models. Some need never work in a lab at all, and instead deal with mathematical equations.
“Physics is becoming more collaborative; it’s not like the old days, where there was a single person in an office, writing down a theory,” said Associate Professor Wei-Cheng Lee, a condensed matter theorist who frequently works with Aynajian. “Today’s physics research involves many different people with different areas of expertise.”
What is a quantum material?
Quantum materials exhibit qualities that typically aren’t seen in simple materials or at room temperature, such as superconductivity. At the heart of this phenomenon are tiny fundamental particles known as electrons, which surround an atom’s nucleus; all electrons have a negative charge and repel one another. Quantum materials are broadly defined as solids with exotic properties due to novel quantum behaviors of interacting electrons.
One example is superconductivity, which has resulted in a number of Nobel prizes in physics.
“A superconductor is a material in which electrons can be attracted to each other; they form pairs of electrons,” Aynajian explained. “When they do this, something very interesting happens. They become ‘dissipationless’ in that they can go through the material without any loss of energy.”
That loss is typically expressed through heat, whether a smartphone or a data center, he explained. In metals, electrons are free to move around, which is why they can carry electricity under a small voltage. Diamond and glass, however, are insulators: to get electrons to move, you would need to apply enormous voltage from outside the material.
In quantum materials with novel topological qualities, physicists discovered topological insulators about two decades ago. While they contain a normal insulator, their surfaces are conductors such as copper or gold. As a result, electrons can move freely only on the surface. Recently, physicists have found more exotic materials that exhibit even stranger properties: the electrons may only move at the edges of the sample through a single atomic line. In others, electrons may only be available at the corners.
The first such materials were predicted by theorists and then discovered and realized by experimental physicists — the inverse of many discoveries, which usually begin with experiments and are then explained by theorists.
Quantum materials offer unique opportunities for advancing several rapidly evolving fields, including quantum computers and sensors, which are far faster than traditional technology. Think of it this way: The computers we use every day rely on electrical current and a binary code of ones and zeros that manipulates the flow of this current. In quantum reality, an electron can be in two places simultaneously or pass through another substance.
The end result: a quantum computer that can complete certain calculations within minutes or hours that would take 10,000 years in a traditional computer. This concept is known as quantum supremacy, and scientists from diverse fields and various research institutes — even teams at Google, Microsoft and IBM — are actively working toward achieving it. Today, these kinds of computers are the size of a full laboratory, Aynajian pointed out; they also need cold temperatures in the milli-Kelvin range.
“When we talk about quantum computers, quantum bits called qubits are not defined as ones or zeros, as in a classical computer,” Aynajian explained. “They are in a superposition of both states. This allows quantum computers to perform many computations simultaneously.”
The role of modeling and theory
For condensed matter physicists, the holy grail is a superconductor that can operate at room temperature. At present, eligible materials would require enormous pressure, which would make them impractical, Kolmogorov acknowledged.
His work lies at the intersection between physics, materials science and computer science; he and Professor Elena Roxana Margine have combined their separate advanced computational methods to predict materials that could work as next-generation superconductors. One possibility lies with materials based on boron and carbon. In their latest research, Kolmogorov and Margine suggested not only particular materials but also a way these materials could be synthesized.
“So effectively, we’re proposing a whole new class of materials that have the potential to be superconductors at high temperatures, and these are very common elements,” Kolmogorov said. “You don’t need anything exotic: It’s just lithium, sodium, boron, carbon. Hopefully, we’ll have some exciting discoveries.”
As a theorist, Lee doesn’t work in a lab or with supercomputers. Instead, he works with the fundamental principles that underlie the discipline — particularly mathematics.
“I’m a theoretical physicist; we use math as a tool,” he said. “At the end of the day, we need to apply the theory to understand something in the real world. Otherwise, it’s not a theory, even if the math is beautiful.”
As he does sometimes in class, Lee held up a red and blue ribbon to explain how topological materials work. Connect it into a loop, and you form one state; twist that ribbon before connecting the ends, and you have another. You can tangle the ribbon and then untangle it, but the fundamental way that it is connected doesn’t change.
These two states cannot continuously transform into one another without breaking the ribbon, which explains—in essence—how electrons could be limited to certain edges and paths in a material that otherwise doesn’t permit their passage. To use purely mental imagery, consider doughnuts and bowls. Both can be considered deformations of a solid sphere, but they are topologically different because doughnuts have a hole, and bowls don’t. In this sense, doughnuts and mugs are topologically equivalent since both have a hole.
“It is really exciting to see this principle of topology could be realized in quantum materials in the real world, and all of these come directly from basic principles of quantum mechanics!” Lee added.
Any of the department’s paths — theory, computational modeling or experiment — may lead to the next great discovery. Every one of these paths is critical to the effort.
“Research at this level cannot be done by a single person,” Aynajian said. “Collaboration between theorists, experimentalists, materials and computational scientists is essential, and there are always many challenges to overcome. But when it finally works, it’s a great discovery.”